Thulium-Doped Fiber Laser and Its Application in Urinary Lithotripsy
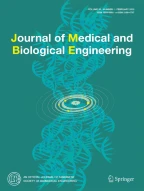
Thulium-doped fiber laser (TFL)-based urological lithotripsy has emerged as a promising technology for treating urological stones in new era of endourological options. The objective of this review is to provide a comprehensive summary of the characteristics of TFL application for lithotripsy and to analyze the research progress of two crucial TFL light sources, quasi-continuous (QCW) TFL and main oscillation power amplification (MOPA) TFL.
Methods
The data about the potential efficiency of TFL in clinical applications and the status of pulsed TFL studies were gathered through a manual search of TFL-related literature in the SCI, SSCI, A&HCI, IE, PubMed databases to assess the performance issues associated with application of thulium laser for urinary tract stone surgery.
Results
TFLs can ablate stones mainly through photothermal and micro-explosive effects. In clinical trials, thulium laser has been established to be superior to holmium laser in terms of lithotripsy efficiency, tissue damage, lithotripsy retreating effect, thermal effect, intraoperative visualization, and postoperative lithotripsy-free rate, which is more widely used currently. Among the various pulsed TFLs, QCW TFL provides flexible beam waveform, high electro-optical conversion efficiency, high heat dissipation performance, and low material maintenance requirements. MOPA TFL can flexibly affect the morphology of the output pulse within a wide range while significantly increasing the output power.
Conclusion
TFL represents a safer, more effective and comprehensive tool for the treatment of urinary stone. Although QCW TFL is currently the mainstream laser source for lithotripsy, we anticipate that MOPA technology has the potential to offer number of advantages while it has not yet received sufficient attention. Further studies are still needed to optimize the performance of pulsed TFLs to facilitate the development of thulium laser lithotripsy.
This is a preview of subscription content, log in via an institution to check access.
Access this article
Subscribe and save
Springer+ Basic
€32.70 /Month
- Get 10 units per month
- Download Article/Chapter or eBook
- 1 Unit = 1 Article or 1 Chapter
- Cancel anytime
Buy Now
Price includes VAT (France)
Instant access to the full article PDF.
Rent this article via DeepDyve
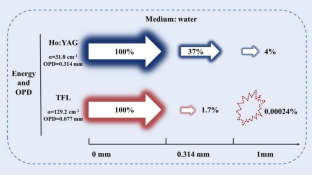
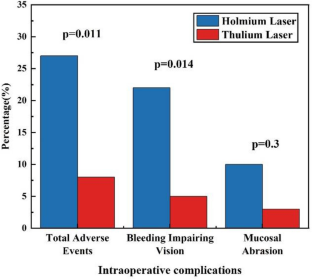
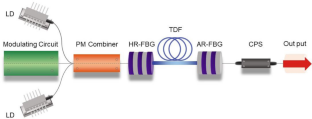
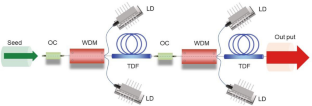
Similar content being viewed by others
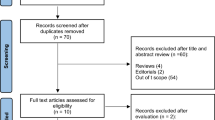
Thulium:YAG laser: a good compromise between holmium:YAG and thulium fiber laser for endoscopic lithotripsy? A narrative review
Article 06 November 2023
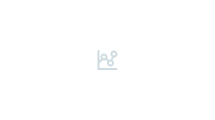
Thulium Fiber Laser Behavior on Tissue During Upper- and Lower-Tract Endourology
Article 30 September 2022
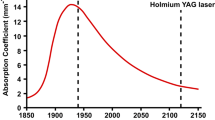
Thulium fiber laser: the new player for kidney stone treatment? A comparison with Holmium:YAG laser
Article Open access 06 February 2019
Data Availability
The authors declare that all data supporting the findings of this study are available within the paper. Additional review data can be shared upon request in Covidence.
References
- Kinn, A. C., Fernstrom, I., Johansson, B., et al. (1991). Percutaneous nephrolithotomy—the birth of a new technique. Scandinavian Journal of Urology and Nephrology,25, 11–14. ArticleGoogle Scholar
- Rassweiler, J. (2006). A landmark paper for endourology. European Urology,50(3), 395–399. ArticlePubMedGoogle Scholar
- Chaussy, C., Schmiedt, E., Jocham, D., et al. (2017). First clinical experience with extracorporeally induced destruction of kidney stones by shock waves. Journal of Urology,197(2), S160–S163. PubMedGoogle Scholar
- Fried, N. M., & Irby, P. B. (2018). Advances in laser technology and fibre-optic delivery systems in lithotripsy. Nature Reviews Urology,15(9), 563–573. ArticlePubMedGoogle Scholar
- Dretler, S. P. (1988). Laser lithotripsy—a review of 20 years of research and clinical-applications. Lasers in Surgery and Medicine,8(4), 341–356. ArticleCASPubMedGoogle Scholar
- Pierre, S., & Preminger, G. M. (2007). Holmium laser for stone management. World Journal of Urology,25(3), 235–239. ArticlePubMedGoogle Scholar
- Lerner, L. B., & Tyson, M. D. (2009). Holmium laser applications of the prostate. Urologic Clinics of North America,36(4), 485–495. ArticlePubMedGoogle Scholar
- Traxer, O., & Keller, E. X. (2020). Thulium fiber laser: The new player for kidney stone treatment? A comparison with Holmium:YAG laser. World Journal of Urology,38(8), 1883–1894. ArticleCASPubMedGoogle Scholar
- Razvi, H. A., Denstedt, J. D., Chun, S. S., et al. (1996). Intracorporeal lithotripsy with the holmium:YAG laser. Journal of Urology,156(3), 912–914. ArticleCASPubMedGoogle Scholar
- Teichman, J. M. H., Vassar, G. J., Bishoff, J. T., et al. (1998). Holmium:YAG lithotripsy yields smaller fragments than lithoclast, pulsed dye laser or electrohydraulic lithotripsy. Journal of Urology,159(1), 17–23. ArticleCASPubMedGoogle Scholar
- Keller, E. X., de Coninck, V., Audouin, M., et al. (2019). Fragments and dust after holmium laser lithotripsy with or without “Moses technology”: How are they different? Journal of Biophotonics,12(4), e201800227. ArticlePubMedGoogle Scholar
- Kronenberg, P., & Traxer, O. (2015). Update on lasers in urology 2014: Current assessment on holmium:Yttrium-aluminum-garnet (Ho:YAG) laser lithotripter settings and laser fibers. World Journal of Urology,33(4), 463–469. ArticlePubMedGoogle Scholar
- Kronenberg, P., & Traxer, O. (2014). The truth about laser fiber diameters. Urology,84(6), 1301–1307. ArticlePubMedGoogle Scholar
- Emiliani, E., Talso, M., Haddad, M., et al. (2018). The true ablation effect of holmium YAG laser on soft tissue. Journal of Endourology,32(3), 230–235. ArticlePubMedGoogle Scholar
- Turk, C., Petrik, A., Sarica, K., et al. (2016). EAU guidelines on interventional treatment for urolithiasis. European Urology,69(3), 475–482. ArticlePubMedGoogle Scholar
- Healy, K., Chamsuddin, A., Spivey, J., et al. (2009). Percutaneous endoscopic holmium laser lithotripsy for management of complicated biliary calculi. JSLS-Journal of the Society of Laparoendoscopic Surgeons,13(2), 184–189. PubMedPubMed CentralGoogle Scholar
- Hardy, L. A., Vinnichenko, V., & Fried, N. M. (2019). High power holmium:YAG versus thulium fiber laser treatment of kidney stones in dusting mode: Ablation rate and fragment size studies. Lasers in Surgery and Medicine,51(6), 522–530. ArticlePubMedGoogle Scholar
- Chan, K. F., Pfefer, T. J., Teichman, J. M. H., et al. (2001). A perspective on laser lithotripsy: The fragmentation processes. Journal of Endourology,15(3), 257–273. ArticleCASPubMedGoogle Scholar
- Hall, L. A., Gonzalez, D. A., & Fried, N. M. (2019). Thulium fiber laser ablation of kidney stones using an automated, vibrating fiber. Journal of Biomedical Optics. https://doi.org/10.1117/1.JBO.24.3.038001ArticlePubMedPubMed CentralGoogle Scholar
- Keller, E. X., de Coninck, V., Doizi, S., et al. (2021). Thulium fiber laser: Ready to dust all urinary stone composition types? World Journal of Urology,39(6), 1693–1698. ArticleCASPubMedGoogle Scholar
- Kronenberg, P., & Traxer, O. (2019). The laser of the future: Reality and expectations about the new thulium fiber laser-a systematic review. Translational Andrology and Urology,8, S398–S417. ArticlePubMedPubMed CentralGoogle Scholar
- Kronenberg, P., Hameed, B. M. Z., & Somani, B. K. (2021). Outcomes of thulium fibre laser for treatment of urinary tract stones: Results of a systematic review. Current Opinion in Urology,31(2), 80–86. ArticlePubMedPubMed CentralGoogle Scholar
- Andreeva, V., Vinarov, A., Yaroslavsky, I., et al. (2020). Preclinical comparison of superpulse thulium fiber laser and a holmium:YAG laser for lithotripsy. World Journal of Urology,38(2), 497–503. ArticleCASPubMedGoogle Scholar
- Hardy, L. A., Vinnichenko, V., & Fried, N. M. (2019). High power holmium: YAG versus thulium fiber laser treatment of kidney stones in dusting mode: Ablation rate and fragment size studies. Lasers in Surgery and Medicine,51(6), 522–530. ArticlePubMedGoogle Scholar
- Martov, A. G., Ergakov, D. V., Guseynov, M., et al. (2021). Clinical comparison of super pulse thulium fiber laser and high-power holmium laser for ureteral stone management. Journal of Endourology,35(6), 795–800. ArticlePubMedGoogle Scholar
- Proietti, S., Rodríguez-Socarrás, M. E., Eisner, B. H., et al. (2021). Thulium: YAG versus holmium: YAG laser effect on upper urinary tract soft tissue: Evidence from an ex vivo experimental study. Journal of Endourology,35(4), 544–551. ArticlePubMedGoogle Scholar
- Ulvik, Ø., Æsøy, M. S., Juliebø-Jones, P., et al. (2022). Thulium fibre laser versus holmium:YAG for ureteroscopic lithotripsy: Outcomes from a prospective randomised clinical trial. European Urology,82(1), 73–79. ArticlePubMedGoogle Scholar
- Lee, H., Ryan, R. T., Teichman, J. M. H., et al. (2003). Stone retropulsion during holmium: Yag lithotripsy. Journal of Urology,169(3), 881–885. ArticlePubMedGoogle Scholar
- Elashry, O. M., & Tawfik, A. M. (2012). Preventing stone retropulsion during intracorporeal lithotripsy. Nature Reviews Urology,9(12), 691–698. ArticlePubMedGoogle Scholar
- Wollin, D. A., Ackerman, A., Yang, C., et al. (2017). Variable pulse duration from a new holmium: YAG laser: The effect on stone comminution, fiber tip degradation, and retropulsion in a dusting model. Urology,103, 47–51. ArticlePubMedGoogle Scholar
- Ventimiglia, E., Doizi, S., Kovalenko, A., et al. (2020). Effect of temporal pulse shape on urinary stone phantom retropulsion rate and ablation efficiency using holmium: YAG and super-pulse thulium fibre lasers. BJU international,126(1), 159–167. ArticleCASPubMedGoogle Scholar
- Petzold, R., Miernik, A., & Suarez-Ibarrola, R. (2021). Retropulsion force in laser lithotripsy—an in vitro study comparing a holmium device to a novel pulsed solid-state thulium laser. World Journal of Urology. https://doi.org/10.1007/s00345-021-03668-8ArticlePubMedPubMed CentralGoogle Scholar
- Dragos, L., Somani, B. K., Etienne, X. K., et al. (2019). Super-pulse thulium fiber versus high power holmium lasers. What about temperature? Journal of Urology. https://doi.org/10.1097/01.JU.0000555251.88486.79ArticleGoogle Scholar
- Taratkin, M., Laukhtina, E., Singla, N., et al. (2020). Temperature changes during laser lithotripsy with Ho:YAG laser and novel Tm-fiber laser: A comparative in-vitro study. World Journal of Urology,38(12), 3261–3266. ArticleCASPubMedGoogle Scholar
- Aesoy, M. S., Juliebo-Jones, P., Beisland, C., et al. (2022). Temperature profiles during ureteroscopy with thulium fiber laser and holmium:YAG laser: Findings from a pre-clinical study. Scandinavian Journal of Urology,56(4), 313–319. ArticleCASPubMedGoogle Scholar
- Andreeva, V., Vinarov, A., Yaroslavsky, I., et al. (2020). Preclinical comparison of superpulse thulium fiber laser and a holmium: YAG laser for lithotripsy. World Journal of Urology,38, 497–503. ArticleCASPubMedGoogle Scholar
- Hardy, L. A., Wilson, C. R., Irby, P. B., et al. (2014). Thulium fiber laser lithotripsy in an in vitro ureter model. Journal of Biomedical Optics,19(12), 128001. ArticlePubMedGoogle Scholar
- Blackmon, R. L., Irby, P. B., & Fried, N. M. (2010). Holmium:YAG (lambda=2,120nm) versus thulium fiber (lambda=1,908nm) laser lithotripsy. Lasers in Surgery and Medicine,42(3), 232–236. ArticlePubMedGoogle Scholar
- Enikeev, D., Taratkin, M., Klimov, R., et al. (2020). Thulium-fiber laser for lithotripsy: First clinical experience in percutaneous nephrolithotomy. World Journal of Urology,38, 3069–3074. ArticleCASPubMedGoogle Scholar
- Korolev, D., Akopyan, G., Tsarichenko, D., et al. (2021). Minimally invasive percutaneous nephrolithotomy with SuperPulsed Thulium-fiber laser. Urolithiasis,49, 485–491. ArticleCASPubMedGoogle Scholar
- Traxer, O., & Corrales, M. (2021). Managing urolithiasis with thulium fiber laser: Updated real-life results-a systematic review. Journal of Clinical Medicine,10(15), 3390. ArticleCASPubMedPubMed CentralGoogle Scholar
- Traxer, O., Sierra, A., & Corrales, M. (2022). Which is the best laser for lithotripsy? Thulium fiber laser. European Urology Open Science,44, 15–17. ArticlePubMedPubMed CentralGoogle Scholar
- Wang, X., Jin, X. X., Zhou, P., et al. (2015). 105 W ultra-narrowband nanosecond pulsed laser at 2 μm based on monolithic Tm-doped fiber MOPA. Optics Express,23(4), 4233–4241. ArticleCASPubMedGoogle Scholar
- Zhang, M., Kelleher, E. J. R., Obraztsova, E. D., et al. (2011). Nanosecond pulse generation in lumped normally dispersive all-fiber mode-locked laser. IEEE Photonics Technology Letters,23(19), 1379–1381. ArticleCASGoogle Scholar
- El-Sherif, A. F., & King, T. A. (2003). High-energy, high-brightness Q-switched TM3+-doped fiber laser using an electro-optic modulator. Optics Communications,218(4–6), 337–344. ArticleCASGoogle Scholar
- Shi, W., Petersen, E. B., Nguyen, D. T., et al. (2011). 220 μJ monolithic single-frequency Q-switched fiber laser at 2 μm by using highly Tm-doped germanate fibers. Optics Letters,36(18), 3575–3577. ArticleCASPubMedGoogle Scholar
- Jiang, M., Ma, H. F., Ren, Z. Y., et al. (2013). A graphene Q-switched nanosecond Tm-doped fiber laser at 2 μm. Laser Physics Letters,10(5), 055103. ArticleCASGoogle Scholar
- Li, H. H., Liu, J., Cheng, Z. C., et al. (2015). Pulse-shaping mechanisms in passively mode-locked thulium-doped fiber lasers. Optics Express,23(5), 6292–6303. ArticleCASPubMedGoogle Scholar
- Sobon, G., Duzynska, A., Swiniarski, M., et al. (2017). CNT-based saturable absorbers with scalable modulation depth for Thulium-doped fiber lasers operating at 1.9 μm. Scientific Reports. https://doi.org/10.1038/srep45491ArticlePubMedPubMed CentralGoogle Scholar
- Ahmad, H., Samion, M. Z., Kamely, A. A., et al. (2019). Mode-locked thulium doped fiber laser with zinc oxide saturable absorber for 2 μm operation. Infrared Physics & Technology,97, 142–148. ArticleCASGoogle Scholar
- Chernysheva, M. A., Krylov, A. A., Kryukov, P. G., et al. (2012). Nonlinear amplifying loop-mirror-based mode-locked thulium-doped fiber laser. IEEE Photonics Technology Letters,24(14), 1254–1256. ArticleCASGoogle Scholar
- Gene, J., Yeom, D. I., Kim, S. K., et al. (2021). Long-cavity mode-locked thulium-doped fiber laser for high pulse energy. Optics and Laser Technology,136, 106739. ArticleCASGoogle Scholar
- Pal, D., Ghosh, A., Sen, R., et al. (2016). Continuous-wave and quasi-continuous wave thulium-doped all-fiber laser: Implementation on kidney stone fragmentations. Applied Optics,55(23), 6151–6155. ArticleCASPubMedGoogle Scholar
- Pal, A., Pal, D., Das Chowdhury, S., et al. (2017). All-fiber laser at 1.94 μm: Effect on soft tissue. In Proceedings of the conference on optical interactions with tissue and cells XXVIII, San Francisco, CA, F Jan 30–31, 2017.
- Pal, A., Pal, D., Das Chowdhury, S., et al. (2019). Interaction of thulium fiber laser with urinary stone: Effect of laser parameter on fragmented particle size and retropulsion. In Proceedings of the conference on optical interactions with tissue and cells XXX, San Francisco, CA, F Feb 02–03, 2019.
- Kwon, I. H., Bae, Y., Yeo, U. C., et al. (2018). Histologic analyses on the response of the skin to 1927 nm fractional thulium fiber laser treatment. Journal of Cosmetic and Laser Therapy,20(1), 12–16. ArticlePubMedGoogle Scholar
- Zhou, R. L., Ren, J. C., Yang, C., et al. (2013). An all fiber 1.94 μm nanosecond pulse laser amplified by highly Tm 3+ -doped silicon fibers. Chinese Physics Letters,30(5), 054208. ArticleGoogle Scholar
- Cheng, X., Li, Z. H., Hou, J., et al. (2016). Gain-switched monolithic fiber laser with ultra-wide tuning range at 2 μm. Optics Express,24(25), 29127–29138. ArticleGoogle Scholar
- Grzes, P., & Swiderski, J. (2018). Gain-switched 2 μm fiber laser system providing kilowatt peak-power mode-locked resembling pulses and its application to supercontinuum generation in fluoride fibers. IEEE Photonics Journal,10(1), 1–8. ArticleGoogle Scholar
- Ouyang, D. Q., Zhao, J. Q., Zheng, Z. J., et al. (2015). 110 W all fiber actively Q-switched thulium-doped fiber laser. IEEE Photonics Journal,7(1), 1–6. ArticleCASGoogle Scholar
- Pal, D., Paul, A., Das Chowdhury, S., et al. (2018). Hybrid pumped gain-switched thulium fiber laser at a high repetition rate. Applied Optics,57(13), 3546–3550. ArticleCASPubMedGoogle Scholar
- Limongelli, J. R., Allee, E., Bieniek, M., et al. (2020). A 564 W QCW thulium fiber oscillator pumped at 793 nm. In Proceedings of the laser congress 2020 (ASSL, LAC), Washington, D.C., F 2020/10/13, 2020. Optica Publishing Group.
- IPG Photonics. (2020). IPG photonics corporation. https://www.ipgphotonics.com/en/products/lasers/quasi-cw-fiber-lasers/2-micron-1/tlm-qcw#
- Liu, J., Xu, J., Liu, K., et al. (2013). High average power picosecond pulse and supercontinuum generation from a thulium-doped, all-fiber amplifier. Optics Letters,38(20), 4150–4153. ArticleCASPubMedGoogle Scholar
- Liu, J., Liu, C., Shi, H. X., et al. (2016). High-power linearly-polarized picosecond thulium-doped all-fiber master-oscillator power-amplifier. Optics Express,24(13), 15005–15011. ArticleCASPubMedGoogle Scholar
- Lu, H. B., Zhou, P., Zhang, H. W., et al. (2013). High-power all-fiberized thulium-doped fiber MOPA. Laser Physics Letters,10(12), 125101. ArticleGoogle Scholar
- Liu, J., Wang, Q., & Wang, P. (2012). High average power picosecond pulse generation from a thulium-doped all-fiber MOPA system. Optics Express,20(20), 22442–22447. ArticleCASPubMedGoogle Scholar
- Swiderski, J., Michalska, M., & Grzes, P. (2019). Mode-locking and self-mode-locking-like operation in a resonantly pumped gain-switched Tm-doped fiber laser. Optics Communications,453, 124406. ArticleCASGoogle Scholar
- Tang, Y. L., & Xu, J. Q. (2012). Hybrid-pumped gain-switched narrow-band thulium fiber laser. Applied Physics Express,5(7), 072702. ArticleGoogle Scholar
- El-Sherif, A. F., & King, T. A. (2003). High-energy, high-brightness Q-switched Tm 3+ -doped fiber laser using an electro-optic modulator. Optics Communications,218(4), 337–344. ArticleCASGoogle Scholar
- Tang, Y., Yu, X., Li, X., et al. (2014). High-power thulium fiber laser Q switched with single-layer graphene. Optics Letters,39(3), 614–617. ArticleCASPubMedGoogle Scholar
- Ahmed, M. H. M., Khaleel, W. A., Sadeq, S. A., et al. (2021). Mode-locked thulium doped fiber laser utilizing tungsten trioxide saturable absorber. Optics and Laser Technology,136, 106730. ArticleCASGoogle Scholar
- Chua, M. E., Bobrowski, A., Ahmad, I., et al. (2023). Thulium fibre laser vs holmium: Yttrium-aluminium-garnet laser lithotripsy for urolithiasis: Meta-analysis of clinical studies. BJU International,131, 383–394. ArticleCASPubMedGoogle Scholar
Acknowledgements
The authors would like to thank all the reviewers who participated in the review, as well as MJEditor (www.mjeditor.com) for providing English editing services during the preparation of this manuscript.
Funding
This work was supported by the National Natural Science Foundation of China (62075200), the Fundamental Research Funds for the Central Universities (2042023kf0113, 2042022gf0004), Key Research and Development Program of Hubei province (2023BCB001), the Translational Medicine and Multidisciplinary Research Project of Zhongnan Hospital of Wuhan University (ZNJC202217, ZNJC202232), the Science Fund for Distinguished Young Scholars of Hubei Province (2021CFA042).
Author information
Authors and Affiliations
- The Institute of Technological Sciences, Wuhan University, #299, Bayi Road, Wuhan, 430072, Hubei, China Runyan Gu, Cheng Lei & Du Wang
- Department of Urology, Zhongnan Hospital of Wuhan University, #169, East Lake Road, Wuhan, 430072, Hubei, China Zhilong Li, Sheng Li & Xinghuan Wang
- Runyan Gu